What are the potential applications of a peroxide-based solution that undergoes a specific electrochemical process? A novel approach to peroxide activation could revolutionize various industries.
The term likely refers to a solution of hydrogen peroxide undergoing a voltage-based activation process, potentially in an electrolytic cell. This electrochemical treatment could change the properties of the hydrogen peroxide, increasing its effectiveness in certain applications. For instance, a stronger oxidizing agent might result from this process, making it more effective for disinfection or cleaning purposes. The exact nature and specifications of the process would determine the specifics, including the voltage levels, type of electrode materials, and other parameters.
The importance of such a process hinges on its potential for enhanced efficacy and selectivity compared to traditional peroxide solutions. If the electrochemical process effectively concentrates or modifies the peroxide's reactive properties, it could be beneficial for applications such as: enhanced bleaching in textile or pulp processing; novel sterilization techniques in medical or food industries; or improved chemical reactions in synthesis or manufacturing. This process could also lead to higher yields and reduced waste byproducts. The precise benefits would depend on the specific applications. Past research on electrochemistry and the use of hydrogen peroxide suggests this approach could open new avenues for industrial solutions and potential applications. Further research into the exact reaction mechanisms and optimizing the electrochemical parameters would help to establish the full potential of this approach.
Moving forward, exploring the chemical kinetics and the potential applications of this electrolytic approach to peroxide activation will be key to understanding its broader impacts. Investigations on different substrates, reaction environments, and scale-up processes are essential to determine its practical viability.
Voltstanding Peroxide
Understanding the key aspects of "voltstanding peroxide" is crucial for evaluating its potential applications. Electrochemical activation of peroxide solutions may offer enhanced efficacy and selectivity.
- Electrochemical activation
- Hydrogen peroxide
- Oxidizing power
- Voltage control
- Reaction kinetics
- Applications
- Substrate compatibility
- Scale-up potential
Electrochemical activation of hydrogen peroxide, controlled by voltage, directly impacts its oxidizing power. Reaction kinetics and substrate compatibility are critical factors influencing efficacy in various applications. Understanding the voltage-dependent properties of this process will unlock its potential in diverse fields, such as industrial bleaching and medical sterilization. The scale-up potential for this process could lead to broader industrial adoption, leveraging efficient and controlled peroxide activation. Successfully scaling up production of this technology will necessitate consideration of the required materials for effective electrolysis and the overall cost-effectiveness for its implementation.
1. Electrochemical Activation
Electrochemical activation, a crucial component of processes involving "voltstanding peroxide," represents the controlled application of an electric current to induce changes in a peroxide solution. This process alters the chemical properties of hydrogen peroxide, often enhancing its oxidizing power. The specific voltage employed ("voltstanding") directly impacts the reaction's outcome, dictating the degree of peroxide activation. This controlled activation process is fundamental to achieving desired levels of efficacy in various applications, such as disinfection, bleaching, and chemical synthesis. For example, precise voltage control during the activation process can lead to enhanced oxidation of targeted pollutants in wastewater treatment, leading to more effective contaminant removal.
The importance of electrochemical activation in the context of peroxide-based applications stems from its ability to tailor the peroxide's reactivity. Traditional methods of peroxide application might involve uncontrolled reactions or lower efficacy. Electrochemical activation offers a more precise and controllable method, optimizing the oxidizing power for specific reactions. Furthermore, this approach potentially reduces byproducts and maximizes the yield of the desired outcome. Consider, for example, the bleaching of textiles; precise electrochemical activation of peroxide can achieve a more efficient and targeted bleaching without causing damage to the fabric fibers, resulting in superior quality products. This is exemplified by advancements in the pulp and paper industry, where controlled peroxide activation enhances the bleaching efficiency of wood pulp, ultimately lowering production costs.
In summary, electrochemical activation is not merely a step in the broader process of peroxide application; it is a fundamental component defining the outcome and efficiency of "voltstanding peroxide." By meticulously controlling the electrical parameters, this approach enables a more targeted and efficient activation of peroxide, leading to enhanced reactivity and significant advantages across various applications. However, the successful implementation of electrochemical activation hinges on understanding the specific reaction mechanisms, optimizing voltage parameters, and considering the inherent limitations of each particular application to ensure successful outcomes.
2. Hydrogen Peroxide
Hydrogen peroxide, a ubiquitous chemical compound, plays a crucial role in various applications, particularly when subjected to electrochemical activation. The term "voltstanding peroxide" implies a specific approach to handling hydrogen peroxide, leveraging its properties through controlled voltage application. Understanding hydrogen peroxide's inherent characteristics is essential for appreciating the potential advantages of this method.
- Oxidizing Power and Reactivity
Hydrogen peroxide possesses potent oxidizing capabilities. This inherent reactivity is the foundation of its utility in diverse applications. However, its reactivity can be unpredictable without proper control. Voltstanding peroxide aims to harness this reactivity in a controlled manner, adjusting the oxidative potential according to specific requirements. In applications like bleaching or disinfection, precise control over the oxidation process through voltage manipulation becomes critical.
- Stability and Decomposition
Hydrogen peroxide is prone to decomposition, particularly in the presence of catalysts or under certain environmental conditions. This decomposition reduces its effectiveness and can lead to unwanted byproducts. The controlled voltage application associated with voltstanding peroxide could potentially mitigate decomposition, allowing for more sustained and predictable reactivity in various processes.
- Specificity of Oxidation
Hydrogen peroxide's oxidation reactions can be quite broad. However, controlling the voltage during activation might allow for more specific reactions. This targeted approach to oxidation could prove beneficial in applications where unwanted side reactions are detrimental. For example, in certain industrial processes, precision oxidation is crucial to avoid damage to materials or byproducts. Voltstanding peroxide potentially targets specific substances for degradation without damaging surrounding materials.
- Environmental Impact
The use of hydrogen peroxide can have environmental implications, depending on the application. The decomposition byproducts and their subsequent impact on the surrounding environment should be considered in applications involving voltstanding peroxide. Further research into the specific reaction mechanisms and the fate of decomposition products associated with this approach is vital to evaluate the environmental profile of this technology.
In essence, hydrogen peroxide's intrinsic properties, combined with the controlled voltage activation of "voltstanding peroxide," offer a framework for enhanced application effectiveness and efficiency. By adjusting and managing the peroxide's reactivity, this approach may lead to solutions with reduced risks and optimized outcomes across various sectors.
3. Oxidizing Power
Oxidizing power is a crucial aspect of "voltstanding peroxide," directly influencing the efficacy and application potential of this process. The controlled voltage application modifies hydrogen peroxide's inherent oxidizing capacity, impacting its effectiveness in various chemical reactions and applications. Understanding this modification is essential to evaluating the process's overall utility and potential advantages.
- Voltage-Dependent Modification
The voltage applied during the "voltstanding" process directly alters the oxidizing power of the hydrogen peroxide. Higher voltages can lead to a more potent oxidizing agent, increasing the intensity of the oxidation process. Conversely, lower voltages can result in a less reactive form, potentially enhancing the selectivity of the reaction and minimizing unwanted side effects. This direct relationship between voltage and oxidation capacity is key to understanding and controlling the overall process.
- Enhanced Reactivity and Selectivity
The modified oxidizing power can lead to enhanced reactivity in target substrates. This enhanced reactivity can translate into increased efficiency in various applications, such as bleaching, disinfection, and chemical synthesis. Additionally, the controlled oxidation process can lead to higher selectivity, allowing for targeted reactions without harming bystander materials or reactants. This targeted reactivity could be particularly important in certain industrial processes to avoid unwanted side effects.
- Reaction Kinetics and Rate Control
The oxidizing power directly influences the rate of chemical reactions. A more potent oxidizing agent generally accelerates the reaction. Precise control over the oxidizing power through voltage manipulation facilitates the control of the reaction kinetics, offering an opportunity to optimize the process for efficiency and yield. The speed at which reactions occur is a crucial consideration when optimizing the procedure and scaling up the process for industrial applications.
- Impact on Byproducts and Efficiency
Oxidizing power also affects the formation of byproducts. Changes in voltage can potentially alter the production of these byproducts, influencing the overall efficiency of the process. A controlled oxidation process can often result in a lower amount of undesirable byproducts and a higher yield of desired products. This reduction in byproducts may lead to more environmentally friendly and economically viable processes.
In conclusion, the controlled modification of oxidizing power through "voltstanding peroxide" is crucial to the process's efficacy and application. Precise voltage control allows for optimized reactivity, selectivity, kinetics, and byproduct management. Understanding these relationships is vital for determining the process's overall potential, enabling targeted optimization for a variety of applications.
4. Voltage control
Voltage control is the cornerstone of "voltstanding peroxide." Precise control over applied voltage directly modifies the oxidizing power of hydrogen peroxide, influencing reaction kinetics and outcomes. This control is critical for achieving desired results in diverse applications. Varying the voltage subtly can dramatically alter the process's efficiency and selectivity. For instance, adjusting voltage during a bleaching process can optimize the oxidation of lignin without degrading cellulose, leading to superior fabric quality. In disinfection applications, tailored voltage may enable selective oxidation of pathogens while minimizing harm to surrounding materials. Furthermore, controlling voltage in industrial synthesis can dictate the formation of desired products while minimizing unwanted byproducts. This control, therefore, represents a powerful tool for optimization.
The practical significance of understanding voltage control in "voltstanding peroxide" is profound. Precise voltage application allows for a targeted and efficient approach to oxidation processes, reducing potential hazards and optimizing yields. By controlling the electron transfer during electrolysis, the desired chemical transformations can be achieved with greater efficiency. Industrial applications such as enhanced pulp bleaching, wastewater treatment, and targeted chemical synthesis directly benefit from this control, potentially reducing costs and improving environmental performance. In medical sterilization, controlled voltage can enhance the effectiveness of peroxide-based disinfectants, potentially improving infection control without causing detrimental side effects. Optimizing voltage control provides a pathway to enhanced effectiveness and broader applicability across diverse fields.
In summary, voltage control is not merely a technical detail in "voltstanding peroxide"; it is the critical factor enabling the targeted and effective use of hydrogen peroxide's oxidizing potential. Understanding the nuanced relationship between voltage and the resultant oxidation is essential for optimizing processes in a multitude of applications. Further research into voltage-dependent reaction mechanisms, including the identification of optimal voltage ranges for different applications, remains crucial. Furthermore, exploring the economic and environmental impacts of optimized voltage control in industrial settings will be crucial to fostering the wider adoption of this technology.
5. Reaction Kinetics
Reaction kinetics, the study of reaction rates, is intrinsically linked to "voltstanding peroxide." The controlled voltage application in this process directly influences the rate at which hydrogen peroxide undergoes chemical transformations. Faster reactions are generally more efficient, particularly in industrial applications. The efficiency and effectiveness of "voltstanding peroxide" depend critically on optimizing the reaction kinetics. Understanding these kinetics enables tailoring the process for maximal output in specific applications. For instance, precise control over the rate of oxidation is essential in pulp bleaching, allowing for efficient removal of lignin without damaging cellulose. Similarly, controlling reaction kinetics in medical sterilization applications ensures effective inactivation of microorganisms without detrimental side effects.
Optimizing reaction kinetics in "voltstanding peroxide" processes entails considering various factors. The voltage applied directly impacts the rate of electron transfer, a key step in many reactions involving hydrogen peroxide. Other factors, such as temperature, the presence of catalysts, and the nature of the substrate being oxidized, also significantly influence kinetics. Understanding these interrelationships is essential for effective process control. For example, identifying and optimizing the temperature range where the reaction rate is highest and the byproducts are minimized will maximize efficiency and minimize waste. Analyzing the reaction mechanisms and determining the rate-limiting steps of the process allows for targeted interventions to enhance reaction speed and product yield. Furthermore, the study of reaction kinetics can inform the design of appropriate reactor systems and optimal operational conditions to ensure successful scaling up for industrial applications.
In conclusion, reaction kinetics are not merely a supporting aspect of "voltstanding peroxide"; they are fundamental to its success. Understanding and controlling these kinetics empowers process optimization and allows tailoring the process for varied applications. This understanding necessitates careful consideration of voltage, temperature, catalysts, and substrate characteristics. By precisely controlling reaction rates, "voltstanding peroxide" can be optimized for maximal efficiency and productivity in various industrial and scientific contexts. However, challenges like optimizing for different substrate types, and scaling up to industrial-scale processes remain areas for ongoing research and development.
6. Applications
The practical applications of "voltstanding peroxide" are directly linked to the controlled oxidation afforded by the electrochemical activation of hydrogen peroxide. This controlled approach offers distinct advantages over traditional peroxide applications, potentially enhancing efficiency and selectivity in diverse sectors. The specific applications hinge upon the targeted modification of hydrogen peroxide's oxidizing power, allowing tailored reactions for various processes.
Diverse industrial sectors benefit from this controlled oxidation. Enhanced pulp bleaching in paper production, for instance, demonstrates the potential for optimized lignin removal without compromising cellulose integrity, resulting in higher quality and potentially lower costs. In wastewater treatment, targeted oxidation of pollutants using "voltstanding peroxide" could prove highly efficient, leading to more effective contaminant removal and reduced environmental impact. Furthermore, advancements in medical sterilization may leverage this process for enhanced disinfection capabilities, providing more efficient pathogen inactivation. Likewise, specific chemical synthesis processes may benefit from the precise control over oxidation reactions offered by "voltstanding peroxide," allowing for higher yields and reduced byproducts. Real-world examples are still limited but highlight the potential for substantial gains in various fields when hydrogen peroxide oxidation is effectively controlled and targeted.
The successful implementation of "voltstanding peroxide" hinges on understanding the specific requirements of each application. Optimization of voltage, reaction conditions, and substrate compatibility remains crucial. Challenges in scaling up the technology for large-scale industrial use and considerations of economic viability need further investigation. The environmental impact of the byproducts generated during the process also requires rigorous assessment and mitigation strategies. While the potential for "voltstanding peroxide" is considerable, comprehensive understanding of its diverse applications necessitates further research, development, and optimization. By addressing the specific needs of different sectors and rigorously testing its performance against traditional methods, the practical value of this approach can be fully realized and translated into effective and sustainable industrial processes across a range of applications.
7. Substrate Compatibility
Substrate compatibility is a critical factor in evaluating the effectiveness and applicability of "voltstanding peroxide." The success of this process hinges on the compatibility between the hydrogen peroxide solution, activated by voltage, and the material being treated. Incompatible substrates can hinder reaction efficiency, leading to reduced effectiveness or even damaging side effects. Understanding substrate compatibility is therefore paramount for optimizing the process for various applications.
- Material Reactivity
Different materials react differently to oxidation. Some materials, like certain types of plastics, may be highly susceptible to degradation by the activated peroxide solution, rendering them unsuitable for treatment. Conversely, other materials might exhibit minimal reactivity, making them ideal candidates for various applications. Assessing the inherent reactivity of a material to peroxide oxidation is crucial to determining its compatibility and to avoiding unwanted side effects.
- Chemical Structure and Functional Groups
The chemical structure and presence of specific functional groups within a substrate significantly impact its compatibility with "voltstanding peroxide." The presence of certain functional groups, for instance, could enhance or inhibit the oxidation process. Understanding these interactions allows for targeted material selection and tailored process optimization to maximize effectiveness. Specific functional groups will exhibit different reactivity patterns, leading to varied results from the activation process.
- Surface Properties
Surface properties like texture and porosity influence the reaction rate and uniformity of oxidation. Materials with rough surfaces may present greater contact areas for the activated peroxide, potentially accelerating the oxidation process. Conversely, smooth surfaces might necessitate longer treatment times for complete oxidation. Understanding surface characteristics allows for adjustments in process parameters to ensure consistent and desired results across different substrates.
- Environmental Conditions and Stability
Factors such as temperature and pH influence the overall reaction and compatibility. Optimal conditions can enhance the oxidation process, ensuring desirable results. However, extreme temperatures or inappropriate pH values can hinder the reaction, decrease efficacy, or even cause damage to the substrate. Considering these environmental factors is crucial for proper process optimization.
In conclusion, substrate compatibility is a critical factor in determining the success and practicality of "voltstanding peroxide" applications. By carefully considering the reactivity, chemical structure, surface properties, and environmental factors related to each substrate, the process can be optimized for specific target materials. This optimization ensures maximized efficiency and minimal negative impacts on the treated materials, unlocking the full potential of this approach across diverse industrial and scientific applications.
8. Scale-up Potential
The scale-up potential of "voltstanding peroxide" is a crucial consideration for its practical implementation. Successful scaling translates research findings from laboratory settings to larger-scale industrial applications. The ability to replicate and amplify the electrochemical activation of hydrogen peroxide is vital for broader adoption and economic viability. This requires addressing challenges related to reactor design, material selection, cost-effectiveness, and operational efficiency to ensure the technology's robustness at increasing production volumes. Real-world examples of similar technologies demonstrate the complexity and importance of scale-up feasibility, highlighting the necessity for extensive engineering analysis and process optimization.
Several factors significantly influence the scale-up potential. Reactor design must accommodate increased volume without compromising the controlled voltage application crucial for consistent peroxide activation. Material selection for the electrolytic cells and supporting infrastructure must ensure durability, cost-effectiveness, and compatibility with the oxidizing environment at larger production scales. Cost analysis is imperative, considering both the capital investment for infrastructure and the ongoing operational expenses. Optimizing energy consumption and minimizing byproducts are critical for long-term economic feasibility. For example, a paper mill considering implementing "voltstanding peroxide" for bleaching must account for the scale-up of the electrolytic reactors to process sufficient wood pulp volume. The efficiency of the scaling-up process directly impacts the overall cost-effectiveness and viability of the new approach within the paper industry. Practical experience demonstrates that simply scaling a laboratory setup without careful engineering design can lead to inefficiencies and economic setbacks.
In summary, the scale-up potential of "voltstanding peroxide" is a critical component of its overall value. Addressing the technical, economic, and operational challenges related to scaling is vital for realizing the transformative potential of this technology. Understanding the limitations and necessities for scale-up ensures that the promising results in laboratory settings can be translated into robust and viable industrial applications. This fundamental understanding enables the successful development and implementation of "voltstanding peroxide" across diverse industries. Further research and development focusing on scalable reactor designs, optimized material usage, and efficient energy consumption are vital for realizing the full economic and environmental benefits of this technology.
Frequently Asked Questions about Voltstanding Peroxide
This section addresses common questions and concerns regarding the use and application of voltstanding peroxide, an electrochemical method for activating hydrogen peroxide.
Question 1: What exactly is voltstanding peroxide?
Voltstanding peroxide refers to the electrochemical activation of hydrogen peroxide using a controlled voltage. This process modifies the properties of hydrogen peroxide, often enhancing its oxidizing power and selectivity, compared to traditional peroxide solutions.
Question 2: What are the key benefits of using this method compared to traditional peroxide applications?
Key benefits include potentially enhanced reaction rates, increased selectivity, reduced byproducts, and improved efficiency. The controlled nature of the electrochemical process allows for better control over the oxidation process and potentially minimized environmental impact.
Question 3: What are the potential applications of voltstanding peroxide?
Potential applications span various industries, including pulp and paper bleaching, wastewater treatment, disinfection, and specific chemical syntheses. The targeted oxidation capabilities offer advantages in these applications by enhancing efficiency and reducing environmental impact.
Question 4: What are the challenges associated with scaling up voltstanding peroxide for industrial use?
Scaling up involves addressing challenges in reactor design, material selection, cost-effectiveness, and operational efficiency. Ensuring consistent voltage application at larger scales and maintaining the desired oxidizing power are key concerns.
Question 5: What are the environmental considerations surrounding the use of voltstanding peroxide?
Potential byproducts must be evaluated for environmental impact. Minimizing the formation of undesirable byproducts and their potential environmental consequences requires careful consideration during process optimization. The potential for increased efficiency and reduced chemical waste must be balanced against potential new environmental concerns arising from the new process.
In summary, voltstanding peroxide offers a potentially advantageous approach to utilizing hydrogen peroxide, but proper understanding of the process, including its limitations and potential implications, is crucial. Further research and development are essential for optimizing the method for different applications and scaling it for industrial use.
This concludes the FAQ section. The following section will delve deeper into the technical aspects of the voltstanding peroxide process.
Conclusion
This exploration of "voltstanding peroxide" reveals a promising electrochemical approach to activating hydrogen peroxide. The controlled voltage application offers the potential for enhanced oxidizing power, reaction selectivity, and reduced byproducts compared to traditional methods. Key factors such as voltage control, reaction kinetics, substrate compatibility, and scale-up potential were meticulously examined. The potential applications in industries like pulp and paper, wastewater treatment, and medical sterilization are significant, suggesting the possibility of considerable gains in efficiency and reduced environmental impact. However, further research and development are necessary to fully optimize the process for diverse applications and overcome scalability challenges. Cost-effectiveness and environmental assessments of byproducts are crucial for determining the broader applicability and long-term viability of this technology.
The future of "voltstanding peroxide" hinges on the successful resolution of technical hurdles and the robust demonstration of economic viability. Further investigations into specific reaction mechanisms, optimized reactor designs, and tailored process parameters for various substrates are imperative. Addressing environmental concerns by carefully examining byproducts and optimizing waste management strategies will be critical for widespread adoption and sustainable implementation. The potential benefits, while substantial, necessitate continued research and development to translate laboratory findings into reliable industrial processes. This comprehensive approach, incorporating a nuanced understanding of the scientific underpinnings and practical challenges, is essential for realizing the full potential of this technology.
You Might Also Like
Beyond Thelma Cora: Uncover Her StoryPremium Snackbox Micro: Delicious Bites For On-the-Go!
Best WAOS Memes & Funny Reactions!
Best BVb Makeup Looks & Trends
Best Brioche In London: Delicious Treats Await!
Article Recommendations
![[OFFICIAL] Voltstanding Tier List is HERE.. Peroxide YouTube](https://i.ytimg.com/vi/-6jQwcmxUv4/maxresdefault.jpg)

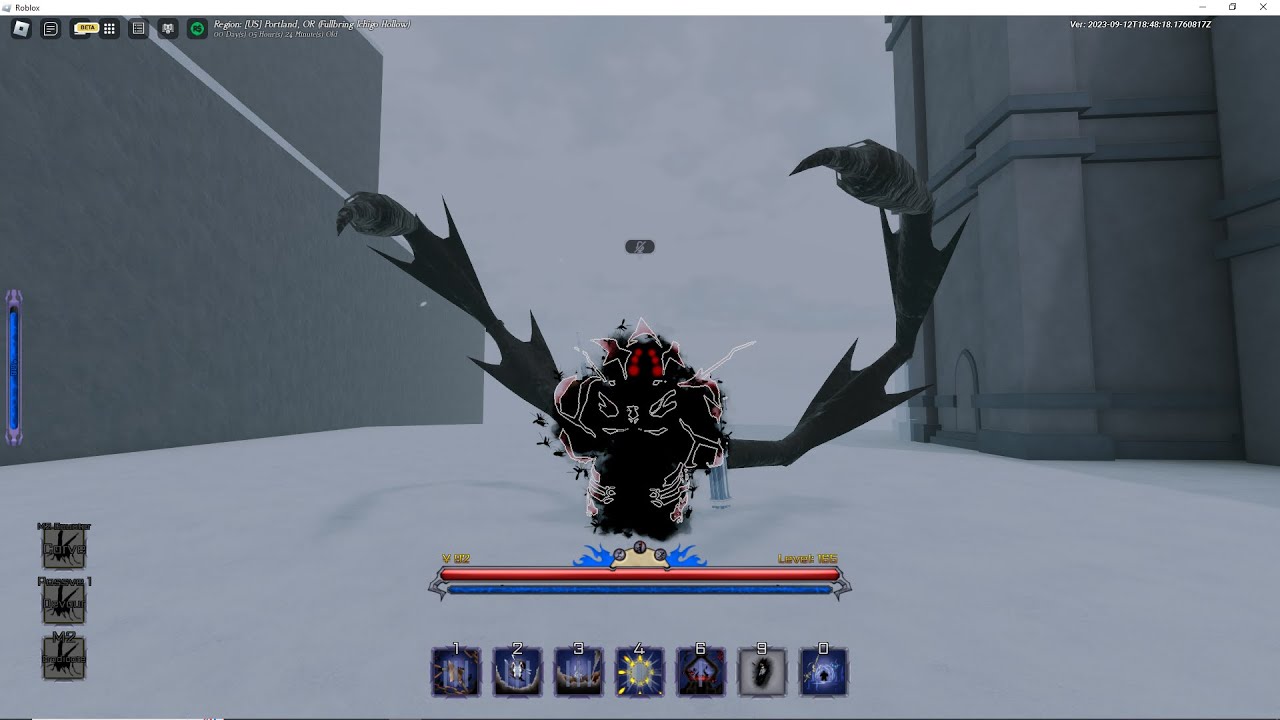